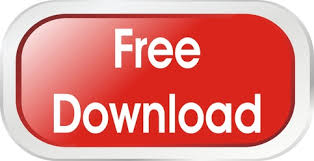
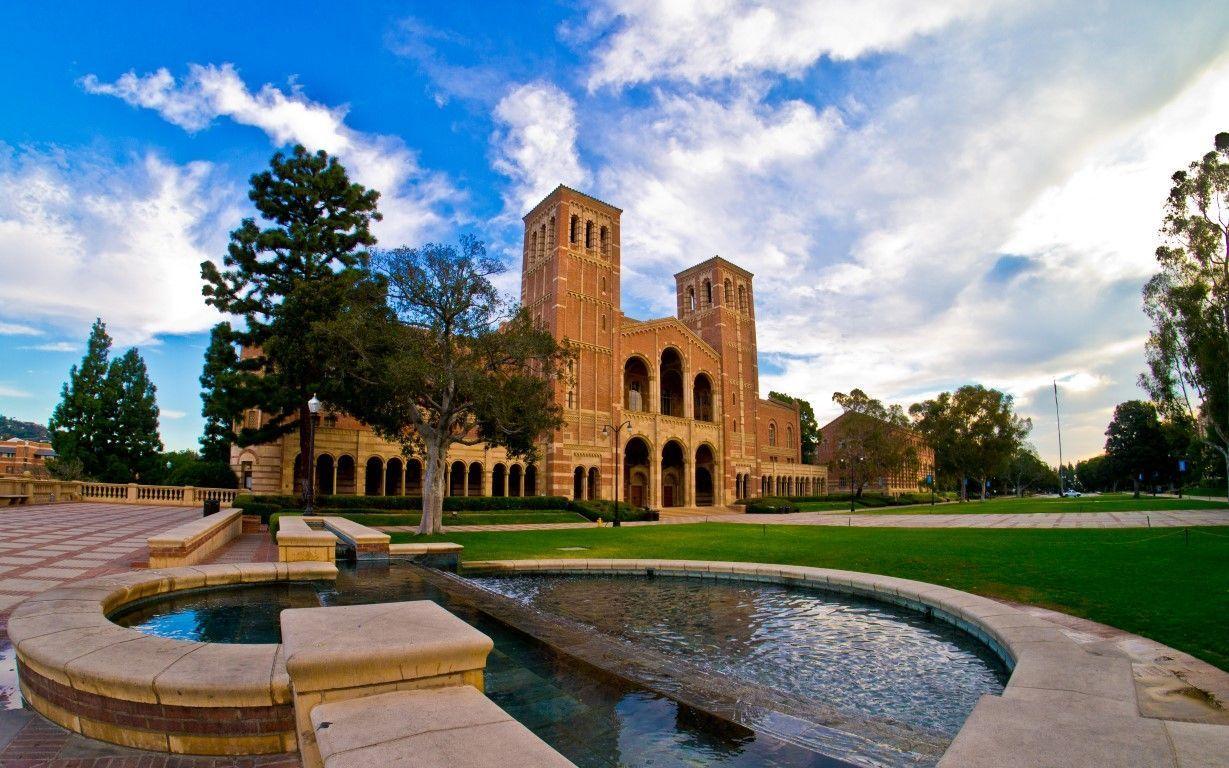
Such lapses in ensuring that the IPv6 network is properly managed and secured are leaving thousands of important devices more vulnerable to attack than before IPv6 was enabled. We found systemic discrepancies between intended security policy-as codified in IPv4-and deployed IPv6 policy. We tested a sample of 25 thousand routers and 520 thousand servers against sets of TCP and UDP ports commonly targeted by attackers.
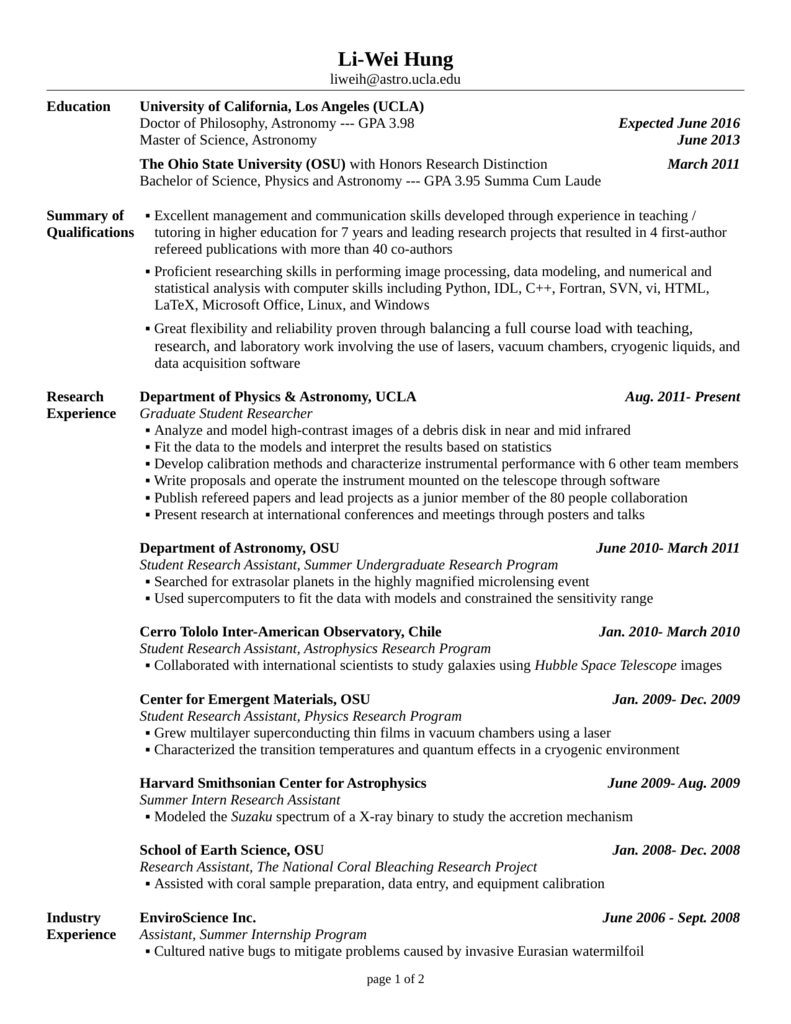
Finally, a third study examines the state of IPv6 network security policy. Observed dark (unallocated destination) IPv6 traffic shows substantial differences from the unwanted traffic seen in IPv4-in both character and scale. This instability is responsible for the majority of the captured misdirected IPv6 traffic. Our analyses suggest that routing of average IPv6 prefixes is less stable than that of IPv4. Next, a network telescope study covering the IPv6 address space of the majority of allocated networks provides insight into the early state of IPv6 routing. Based on cross-dataset analysis of relative global adoption rates and across features of the protocol, we find evidence of a marked shift in the pace and nature of adoption in recent years and observe that higher-level metrics of adoption lag lower-level metrics. The first study provides an analysis of ten years of IPv6 deployment data, including quantifying twelve metrics across ten global-scale datasets, and affording a holistic understanding of the state and recent progress of the IPv6 transition. The work includes three studies, each the largest of its kind, examining various facets of the new network protocol's deployment, routing maturity, and security. Via Internet-scale experiments and data analysis, this dissertation characterizes the adoption and security of the emerging IPv6 network. Recent IPv4 address exhaustion events are ushering in a new era of rapid transition to the next generation Internet protocol-IPv6. Our data collection and topology generation process have been automated, and we publish the latest topology on the web on a daily basis.

The resulting topology graph on a recent day contains 44% more links and 3% more nodes than that from using RouteViews routing tables alone. Second, in addition to using routing tables, we also accumulate topological information from routing updates over time.

First, in addition to using data from RouteViews and RIPE RIS, we also collect data from many other sources, including route servers, looking glasses, and routing registries. In this work, we assemble the most complete AS-level topology by extending the conventional method along two dimensions. Conventionally this topology graph is derived from routing tables collected by Route Views or RIPE RIS. This AS-level topology graph has been widely used in a variety of research efforts. At the inter-domain level, the Internet topology can be represented by a graph with Autonomous Systems (ASes) as nodes and AS peerings as links.
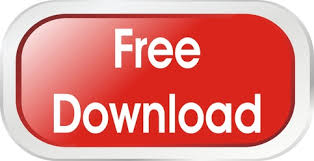